TCI Anesthesia: New and More Universal Models
Natalie Samuda. RM, BSc. Becton Dickinson. Senior Clinical Resource Consultant. Medication Management Solutions. MENAT.
James Waterson. RN, M.Med.Ed. MHE. Becton Dickinson. Medical Affairs Manager, Middle East & Africa.
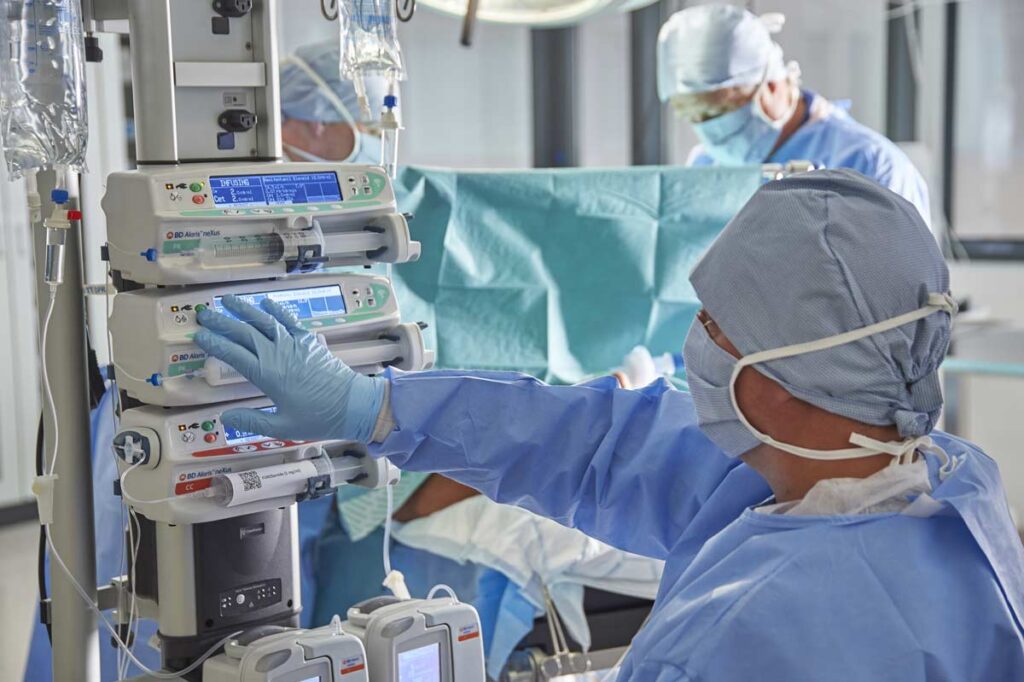
An Evolving Science
Total intravenous anesthesia (TIVA) has been a viable technique since the synthesis of the first intravenous anesthetics. From the introduction of barbiturates in 1921 and Thiopental in 1934, TIVA has continued to develop. Research in the area really took off however after the launch of the fast-acting hypnotic Propofol by Astra Zeneca in 1977.
It is important to define clearly between TIVA and Target-Controlled Infusion (TCI). TIVA means nothing more than anesthesia is being provided to the patient solely via the IV route and that no gas agents are being delivered. The technique known as IVA is a combination of IV drugs and inhalation anesthesia.
TCI was part revolution and part evolution. The first pharmacokinetic (PK) model for the use of TCI was described by Schwilden in 19811 but research had been in undertaken from 1968 onwards. Essentially TCI refers to maintaining the desired plasma or effect site concentration of a drug using an infusion pump managed by a computer, and PK and pharmacodynamic (PD) models. The drug models and the clinical trials that develop them are vital components of the TCI technique, and advances in pump technology have made the technique a common anesthesia technique for the practitioner and have taken it far beyond being just a research tool for specialists.
In simple terms, instead of setting a rate (ml/h) rate or a dose rate (µg or mg/kg/h) on the pump, the pump is programmed to target a required plasma concentration or effect-site concentration. A TCI pump automatically calculates how much drug is needed as induction and maintenance to maintain the desired effect-site or plasma concentration.
The Theory of TCI
In TCI, drugs are delivered to achieve specific predicted target blood or effect site drug concentrations. TCI gives us a standardised infusion system for the administration of hypnotic (Propofol) and analgesic drugs. But how were these blood/effect drug concentrations and pharmacokinetic/pharmacodynamics parameters derived, and how are the models for plasma and effect-site concentrations incorporated into the pump which will control induction and subsequent rate of delivery?
In simple terms a TCI algorithm (the ‘target’ and plan on which the pump relies to deliver appropriate induction and maintenance rates to maintain anesthesia without overdosing the patient) is based on four processes that occur following injection of any intravenous drug into the body:
- Absorption.
- Distribution.
- Metabolism.
- Excretion.
All of the above are commonly, but not always, affected by weight, and of course renal and hepatic health will affect metabolism and elimination. The differences between adults and children in terms of the three processes will be looked at later with a brief review of the specific pediatric TCI models that are currently available.
PK models are derived from pharmacokinetic studies involving heterogeneous volunteers from across a wide spectrum of physiological variables- height, weight, age, and gender. Blood draws are correlated against the clinical endpoint of ‘awareness’ or depth of anesthesia assessed through EEG monitoring or Bispectral Index (BIS) monitors (See Figure 1) and clinical inference using standard observation tools such as the Modified Observer’s Assessment of Alertness/ Sedation Scale (MOAA/S) (See Figure 2)
Figure 1: BIS Scores versus clinical state.
BIS Score. Clinical State.
- 0 Flat Line EEG.
- 20 Burst Suppression.
- 40 Deep Hypnotic Sleep.
- 60 General Anesthesia.
- 80 Light Moderate Sedation.
- 100 Awake
Figure 2: The Modified Observer’s Assessment of Alertness/ Sedation Scale (MOAA/S).
Responsiveness
- Agitated 6
- Responds readily to name spoken in normal tone. (‘Alert’) 5
- Lethargic response to name spoken in normal tone. 4
- Responds only after name is called loudly and/or repeatedly. 3
- Responds only after mild prodding or shaking. 2
- Does not respond to mild prodding or shaking. 1
- Does not respond to deep stimulus. 0
Examples of common PK study results for Propofol and Remifentanil are:
In an un-premedicated patient, the effect-site concentration of Propofol required to produce loss of consciousness is about 3 to 6 µg/ml, depending on the patients’ demographics. Volunteers waking from anesthesia generally have a blood concentration of around 1- 2 µg/ml, though this is dependent on other drugs given during anesthesia. Adequate analgesia with Remifentanil is achieved with 3-6ng/ml. A Remifentanil infusion of 0.25-0.5 µg/kg/min in an ‘average’ man- 70kg, 170cm, 40 years old- produces a blood concentration of around 6ng/ml after 25 minutes.
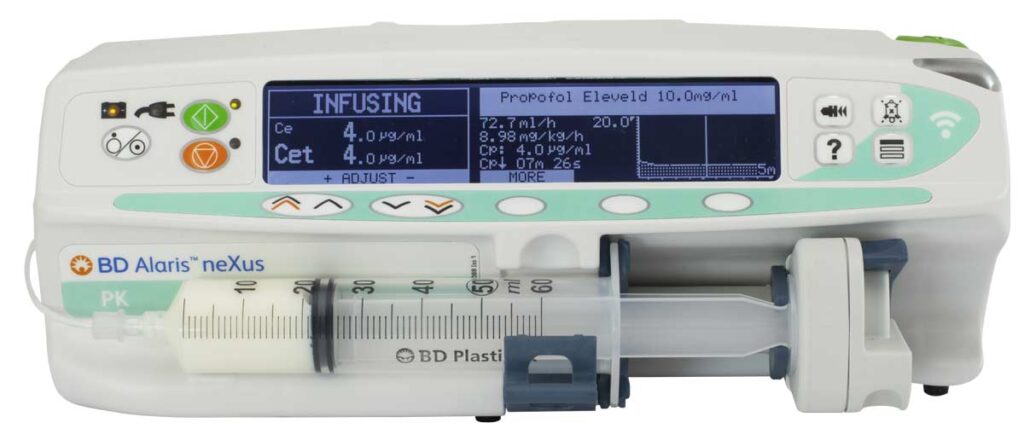
Although various models for propofol were published before, Astra Zeneca decided to implement the pharmacokinetic-dynamic model published by Marsh et al. in their Diprifusor2. This ‘classic’ study is an ideal example to show how a mathematical-pharmacokinetic model is derived from volunteer trials.
The Marsh study and model uses a three-compartment model. ‘Compartments’ relate to theoretical body ‘spaces’ in which a drug will be distributed following injection. Conventionally the body compartment that the drug is injected into is V1 or Vc (plasma/blood), the next compartment is the ‘vessel-rich’ or ‘fast re-distribution’ compartment and is characterized as V2 (heart, liver etc.). The final compartment, which is anatomically ‘vessel-poor’ and ‘slow’ in terms of re-distribution, is V3.(fatty tissue)
Once a steady state of drug distribution has occurred V1+V2+V3=Vdss where Vdss is the steady-state volume of distribution of the drug.
Of course drug distribution and the metabolism/elimination of each drug in each compartment also need to be modelled. By convention the rate of elimination of a drug is K10, whilst the movement/distribution between compartments is denoted by K12 (V1 to V2), K21 (V2 to V1), K13 (V1 to V3) and K31 (V3 to V1). If one wants to describe the hysteresis between the time course of plasma concentration and clinical effect, the pharmacokinetic model must be enlarged with a pharmacodynamic part. The link between the plasma and the effect-site is done by using the time constant ke0. The above provides only a brief overview but assists us when we are reviewing the literature pertaining to the original TCI models, developments built on the original ‘classics’ and papers that address the future potential and current limitations of the technique.
Computer simulations and mathematical modelling of infusion schemes based on the above theories of compartments and clearances give us our models for both Target Plasma Concentration (Cpt) and Target Effect Concentration (Cet) and these can be incorporated into specialist computerised infusion pumps.
The TCI ‘Classics’ and current developments
The pharmacokinetic model most anesthetists may be familiar with is the Marsh model for Propofol 1% and 2%. The model requires age and weight to be programmed in the pump, but does not, in fact, incorporate age into its calculation.
The Schnider model is an alternative model for Propofol 1% and 2% and has advantages in elderly patients as it is based on a lean body mass (LBM) calculation for each patient derived from patient height, and total body weight. The pump calculates doses and infusion rates according to the LBM. It also uses age, height, and gender as factors in addition to weight. Elderly patients, with their lower LBM, therefore receive a lower induction and maintenance dose to maintain a constant plasma concentration. It has been shown that when used in combination with the TCI Remifentanil Minto model a lower effect site concentration is required to induce loss of consciousness.3
One major difference between the Marsh model and the Schnider4,5 model is the assumed size of the V1 compartment. For a 70 kg patient the Marsh model calculates V1 as 15.9 L whereas the corresponding value for Schnider model is 4.27 L. Because of this the estimated concentrations following a bolus or rapid infusions vary greatly. When Propofol administration is stopped, large differences in the estimated concentrations are again found, with the Schnider model estimating a much more rapid fall in concentration than the Marsh model.
Up to now the most commonly used pharmacokinetic model for Remifentanil is the Minto model6, a three-compartment model that uses age, height, gender and weight, and determines LBM for its calculations. Remifentanil is an ultra-short acting opioid (Half-life of 3 minutes) and this allows prolonged infusions without drug accumulation.
The Alfentanil Maitre model is a weight, age and gender adjusted three-compartment model.7 Interaction studies between this model and Propofol8 have shown that a plasma level of 500µg/ml of Alfentanil corresponds with a much reduced Propofol plasma level being required to ensure loss of consciousness. Knowledge of interactions such as this is vital for optimising the day-today use of TCI.
The Gepts-Sufentanil9 model is commonly used in cardiac surgery and has been found to be accurate even in obese patients.10 Bariatric patients, and their response to opioids and sedatives, is an area of ongoing work in TCI.
Until now separate models have been required for pediatric patients as there are distinct physiological differences between children and adults and this affects the PK of both sedatives and analgesics. A pediatric patient’s V1 is proportionally far larger than that of an adult. This then requires a far higher induction dose per kg body weight. Furthermore, due to higher heart rates, higher organ perfusion and higher metabolism children have a higher cardiac index, drugs therefore move far faster into V2 from V1. A higher maintenance dose per kg body weight is also therefore required, particularly in the age 1-2 years group.
The decrement time in children can also be expected to be longer because drugs accumulate in other body compartments other than in the plasma to a greater degree than in adults. In this respect the ‘context sensitive half-life’ of a drug becomes very significant, this is the relationship between the half-life of a drug and the length of time of infusion. In simple terms the longer a drug runs for, the longer the half-life and the longer the decrement time.
Children less than 1 year old differ significantly from older children in terms of their metabolic pathways, and their ability to eliminate drugs is markedly inferior. Although special models for neonates have been published, these have not been implemented into TCI.11,12
The Propofol-Paedfusor Model13,14 can be used for children over the age of 1, and with a body weight of greater than 5 kg. Initially, low plasma targets are recommended at the outset of anesthesia with increases then being made according to the patient MOOA/S response. The Propofol (1% and 2%) three-compartment Kataria model15 has been used for children from age 3-11 years and 15 kg upwards. It uses plasma targeting and no effect site data is available at present. As with all pediatric TCI models experience and expertise in the specifics of pediatric anesthesia is required of the practitioner.

What is new?
The Eleveld model for both Propofol and Remifentanil is essentially a mathematical ‘synthesis’ of the data from multiple previous studies and model builds. It also has the added feature of adjusting the expected patient response to Propofol in the presence of Remifentanil. The key advantage of this model is that it is near universal and simplifies and streamlines the number of different models in use by the anesthesia department- this makes for better risk management and makes training more focussed. In a recent study16 the model showed a better median prediction of actual measured propofol plasma concentration than could be achieved with the Marsh or Schnider models. The Eleveld model captures patients from age 5 days to 85 years old and with weights between 2.5 kg and 106 kg.
The Kim-Obara-Egan Remifentanil model was developed in 2017 and has been used in patients with age and weight ranges of 20-85 years and 45-215 kg. Clinically, it acts in a similar manner to the Minto model.17
The Hannivort-Colin Dexmetomidine Model is a very interesting development for a medication that is growing in its use and application across critical care. The model has Cpt values and the MOAA/S scale is used rather than BIS, along with clinical assessment of the patient to guide therapy. Achieving steady state anesthesia takes a comparatively long time of 10 minutes, and a Cpt of about 1.5 ng/ml would be expected to result in light sedation. The model has not however been tested on pediatrics or bariatric patients but there is ongoing work to bring a Cet model and assessment by BIS into clinical usage.18
Delivering TCI: Pump Attributes.
A TCI pump consists of a user-interface to enter patient details and target plasma or effect-site concentration, a software package with pharmacokinetic/pharmacodynamic models to control the infusion rate and hardware to accurately deliver the infusion. The patient’s expected drug concentration is continuously calculated by the pump, and pump infusion rates are almost continuously adjusted, typically at 10-second intervals.
The anesthetist selects the model and concentration of drug to be used. They then program the desired target Cpt or Cet concentrations, and input patient data: weight, age, gender and height (depending on the model). The anesthetist may lengthen the induction time for patients who have a fragile cardiovascular status, or start at lower concentrations and slowly induce.
TCI pumps are of course delivering one infusion at a constantly altering rate (in fact they decrease the rate slightly every 8 seconds). But it can be useful to think of this one infusion as being a mean-average comprised of three continually calculated infusion rates: a constant rate to replace drug elimination and two exponentially decreasing infusions to match drug removed from central compartment to other peripheral compartments of distribution.
The pump will also predict a decrement time- this is the time that it takes for the pump’s plasma/effect site predictions to go from the current level to the decrement concentration level. This of course is also a dynamic value and is constantly recalculated by the pump as the length of anesthesia and any changes to target concentrations are changed.
Key features of an ideal TCI infusion system or pump are:
- A large, clear display that is easy to navigate during the many critical phases of anesthesia and easily viewed from a distance when multiple devices surround the patient during surgery.
- Critical information such as decrement time, current Cet or Cpt and respective targets, current dose rate and concentration and type of agent being infused can be displayed at the same time on one screen.
- Patient parameters used during the setting-up of infusions appear on one screen to avoid the need for shuttling through multiple screens to check vital information.
- An option for using pediatric TCI models.
- The ability to edit and customise the programs used. For example to allow for the most commonly used drugs to be at the head of every loaded formulary, to have dedicated profiles for individual anesthetists and to be able to load research agents or new medications into the pump’s drug library. The ability to set default values and safety limits for the parameters that TCI commonly require to be loaded in order to calculate induction and maintenance doses. For example if adult patients are being anesthetised it is ideal if the weight default is set at 75 kg, age to 50 years and height at 180 cm. This saves a great deal of time for the anesthetist in a busy Operating Theatre.
- An Induction Time adjustable from seconds to minutes to allow for a gentle induction for patients with cardiovascular conditions or established hypotension.
- An ability to automatically pause after induction to allow for assessment of the patient.
- A capacity for a wide range of Dose and Rate units including nanogram dosing, particularly when using the TIVA mode of the pump.
- A capacity for setting default dose rates, patient weight, height and age to match the common patient population that the individual anesthetist works with.
- The capacity to have multiple ‘profiles’ loaded on one pump to allow for tailoring of the drugs and models for individual anesthetists’ needs and for specialist patient / procedure requirements.
- The ability to use a Predictive TIVA mode. Predictive TIVA can be used with drugs that have an associated TCI model, but the mode allows the user to select infusion rates and to administer bolus doses as required. The PK model is used to estimate the plasma and effect site concentration and to calculate the decrement time. An ideal TCI Pump will show a graphical representation of the ‘background’ TCI model and current patient Cet or Cpt.
Practicalities and Practical Concerns.
TIVA and TCI allow full anesthesia to take place with just two TCI pumps. The most common combination being a Propofol model and Remifentanil model. Specialist lines should be used with integrated anti-syphon valves (to prevent accidental infusion/injection during syringe changeover) and back-check valves to ensure infusions are delivered to the patient during high rate induction without back-flow occurring in other lines. These lines should also include a back-check valve protected access port for IV fluids or other replacement. (See Images II and III)
Interaction does occur between TCI modelled drugs. For example Propofol and Remifentanil have been reported to show a 41% decrease in volume of distribution when used together (See the note above on the Schnider and Eleveld model). Users should consider reducing effect–site or plasma targets where interactions are known to exist or in patients with known health concerns.
Of course, patient pharmacokinetics and pharmacodynamics vary with age, cardiac output, co-existing disease, concurrent drug administration, body temperature as well as the weight of the patient. These factors play an important role in choosing target concentrations and induction rates.19 Whilst all TCI models have default plasma or effect targets the anesthetist must assess the likely needs of his patient. To this end there has been a drive towards harnessing TCI to continuous Bispectral Index monitoring for all patients.20,21
Training systems that utilise simulation have been available since the late 1990s.22 They are useful in that they can allow trainees to simulate specific patient responses to differing doses and also to situations such as large-scale patient bleeding during anesthesia. Extensive patient bleeding requires swift interventions during TCI- specifically the reduction of targets for sedatives as their plasma concentrations will increase with dropping blood volume, thereby increasing the depth of anesthesia and potentially causing further hypotension to the detriment of the patient’s circulation and oxygenation. This can act as a ‘vicious cycle’ as this reduction in oxygenation causes further accumulation of the sedative agent in the patient’s compartments.
TCI systems have not been extensively studied in terms of their impact on patient comfort, time to discharge home, and reduction in post-operative side-effects such as nausea but the advantages of ‘gas-free’ anesthesia appear obvious and TCI, with its emphasis on evidence-based care and management and its new models seems primed to change our approach to the management of patients receiving sedatives and analgesic agents.
References.
- Schwilden H. A general method for calculating the dosage scheme in linear pharmacokinetics. Eur J Clin Pharmacol 1981; 20:279-289.
- Marsh et al.: Brit J Anesth 1991, 67, 41-48.
- Struys et Al. Comparison of plasma compartment versus two methods for effect compartment-controlled target-controlled infusion for Propofol. Anesthesiology, 2000, 92, 399-406.
- Schnider et Al. The influence of method of administration and covariates on the pharmacokinetics of Propofol in adult volunteers. Anesthesiology, 1998, 88, 1170-1182.
- Schnider TW, Minto CF, Shafer SL et al. – The influence of age on Propofol pharmacodynamic. Anesthesiology, 1999, 90, 1502-1516.
- Minto, Schnider and Shafer. Pharmacokinetics and pharmacodynamics of remifentanil. II. Model application. Anesthesiology 1997, 86, 24-33.
- Maitre et Al. Population pharmacokinetics of alfentanil: The average dose–plasma concentration relationship and interindividual variability in patients. Anesthesiology, 1987, 66: 3–12.
- Vuyk et Al. Alfentanil modifies the pharmacokinetics of Propofol in volunteers. Anesthesiology. 1997, 87:A300.
- Gepts et Al. Linearity of pharmacokinetics and model estimation of sufentanil. Anesthesiology, 1995, 83:6, 1194–1204.
- Barvais et Al. Pharmacokinetic model-driven infusion of sufentanil and midazolam during cardiac surgery: Assessment of the prospective predictive accuracy and the quality of anesthesia. J Cardiothorac Vasc Anesth 2000,14, 402-408.
- Allegaert K, de Hoon J, Verbesselt R et Al. Maturational pharmacokinetics of single intravenous bolus of propofol. Pediatr Anesth 2007; 17: 1028-34
- Allegaert K, Peeters MY, Verbesselt R, et Al. Inter-individual variability in propofol pharmacokinetics in preterm and term neonates. Br J Anesth 2007; 99: 864-70).
- Absalom et Al. Accuracy of the ‘Paedfusor’ in children undergoing cardiac surgery or catheterization. Br J Anesth. 2003, 91:4, 507-13.
- Absalom et Al. Paedfusor pharmacokinetic data set. British Journal of Anesthesia, 2005, 95:1, 110.
- Kataria et Al. The Pharmacokinetics of Propofol in Children Using Three Different Data Analysis Approaches. Anesthesiology. 1994, 80, 104-122.
- Hüppe T, Maurer F, Sessler DI, Volk T, Kreuer S. Retrospective comparison of Eleveld, Marsh, and Schnider propofol pharmacokinetic models in 50 patients. Br J Anesth. 2020 Feb;124(2):e22-e24.
- Kim TK, Obara S, Egan TD. Disposition of remifentanil in obesity: a new pharmacokinetic model incorporating the influence of body mass. Anesthesiology 2017; 126: 1019e32
- Colin PJ, Hannivoort LN, Eleveld DJ, Reyntjens KMEM, Absalom AR, Vereecke HEM, Struys MMRF. Dexmedetomidine pharmacokinetic-pharmacodynamic modelling in healthy volunteers: 1. Influence of arousal on bispectral index and sedation. Br J Anesth. 2017 Aug 1;119(2):200-210. doi: 10.1093/bja/aex085. PMID: 28854538.
- Coppens et Al. Influence of administration rate on Propofol plasma-effect site equilibration. Anesthesiology. 2007, 107, 386-396.
- Gan et Al. BIS Utility Study Group, bispectral index monitoring allows faster emergence and improved recovery from Propofol, Alfentanil, and Nitrous Oxide anesthesia. Anesthesiology. 1997, 87, 808–815.
- Struys et Al: Clinical usefulness of the bispectral index for titrating Propofol target effect–site concentration. Anesthesia. 1998, 53, 4–12.
- Shafer et Al. Testing computer-controlled infusion pumps by simulation. Anesthesiology. 1998, 68, 261–266.